March 28th, 2023
Author: Isha Kaushik
Editor: Manish Verma
“We are the cosmos made conscious and life is the means by which the universe understands itself” – Brian Cox
What if we aren’t real? Perhaps it’s all an imagination. None of us really exist.
An attempt to escape our real-world problems funnily brings almost every person in this universe to question reality. As much as I’d like to mention that after giving hours of pondering on this subject and understanding nearly nothing before giving up and getting back to our normal routines, scientists around the world still ride the trillion-dollar question of whether quantum mechanics can solve the mystery of consciousness and help us arrive at the conclusion of what drives our realities.
In most current mainstream biophysics research on cognitive processes, the brain is modelled as a neural network obeying classical physics. Arguably, that is why we still cannot explain the rise of consciousness. Some researchers believe that laws of classical physics alone cannot handle the objective descriptions of this mirage created by sophisticated neural mechanisms. If they are correct, Quantum mechanics could offer an explanation to its unusual properties. For all, the brain is made of neurons, and like any other cell, neurons require proteins and a wide range of chemicals to function. Given that quantum effects occur at the molecular level, it is conceivable that they could have a significant influence on consciousness.
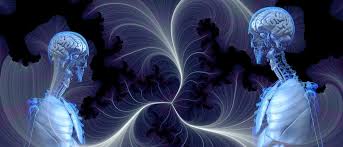
One aspect of the explanation lies in the theory of superposition, the fact that from subatomic to molecular scales, systems can exist in many quantum states at once and with all the mathematical computational machinery in place, the exact position of an electron can be accurately described after the collapse of wave function.
So, what if our thoughts exist in a quantum superposition in an unconscious level in which case, a specific selection- akin to measurement of electron’s position- would give rise to a conscious state. This was proposed by Nobel Prize winning physicist Roger Penrose and anesthesiologist Stuart Hameroff in early 1990’s.
The theory posits that consciousness emerges from the gravitational instabilities within the underlying fabric of space-time. These instabilities cause the collapse of quantum wave functions within microtubules, small proteins located within neurons. These microtubules are believed to function as a quantum computer, optimizing both the performance of individual neurons and their interconnectivity. Presently, scientists at Utrecht University are conducting research on quantum transport within intricately designed fractal structures. (also hypothesized by the Penrose- Hameroff theory).
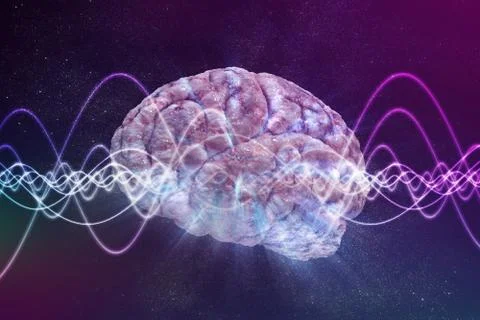
Could unimaginably long distances between neuronal networks get suddenly reduced to negligible, so the entire network could act as one? Might as well.
This is inspired by the theory of quantum entanglement, the ability of two or more quantum systems to establish links between themselves that are sustained across long spatial distance. This link leads them to behave as a single entity.
While there is no actual information being exchanged between the electrons in quantum entanglement, the two systems are nonetheless inextricably linked. This is a special case of superposition. In essence, this means that if we measure the spin (or quantum state) of one of them, let’s say it is spin up, we will instantly know, without measuring it, what the spin of the other electron will be—assume let’s it is in a spin down position. The relevance of this lies in the edge that quantum computing has over classical computing in terms of information processing capacity as long as qubits can uphold their coherence and entanglement. Based on this, some scientists claim that the brain may have figured out how to harness quantum entanglement to perform its extraordinary cognitive computations.
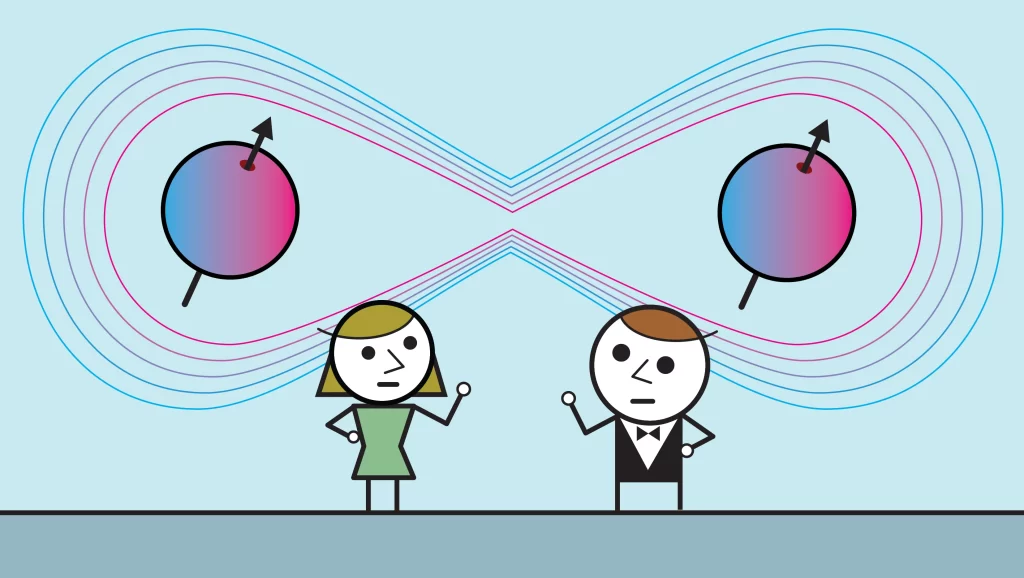
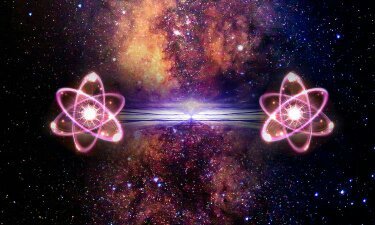
Recently, researchers at Trinity College Dublin hypothesized that entanglement might be at play in human brains using a method to test for quantum gravity. If their results are confirmed, they could be a big step toward understanding how our brain, including consciousness, works.
It’s a great bubble created by some and busted by others like MIT physicist Max Tegmark who suggest that the brain is too busy and warm an environment to sustain coherent quantum states. Quantum- mechanical laws are usually only found to apply in very low temperature conditions (Quantum computing currently operates at -272° Celsius while our body works at room temperatures). In effect, the warm brain environment can turn quantum mechanics into classical physics, which we rely upon currently to produce experimental results on brain functioning.
Quantum mechanics has evolved a very cool mathematical machinery for subatomic predictions over the decades, but with all its weirdness and our inability to relate to wave functions, physicists still can’t explain what it may telling us about the nature of reality. So, the question is that how can one poorly interpreted subject explain another sort of mystery?
Maybe it’s all a myth, or maybe a combination of both the classical and quantum can lead to a conclusion. With all the technological advancement achieved, we are optimistic to be able to pose a solution to this never-ending debate soon, as I too, am on my way back to reality.
REFERENCES
- Stuart Hameroff. (1998). Quantum computation in brain microtubules? The Penrose–Hameroff ‘Orch OR ‘model of consciousness Phil. Trans. R. Soc. A.3561869–1896.
- Albert Thomas Fromhold, Quantum Mechanics, Editor(s): Robert A. Meyers, Encyclopaedia of Physical Science and Technology (Third Edition), Academic Press, 2003, Pages 359-408, ISBN 9780122274107.
- PRYCE, M., SIMON, F. Quantum Mechanics, and Low-Temperature Research. Nature 166, 887–888 (1950).
- Xu, XY., Wang, XW., Chen, DY. et al. Quantum transport in fractal networks. Nat. Photon. 15, 703–710 (2021).
- Christian Matthias Kerskens and David López Pérez 2022 J. Phys. Commun. 6 105001.
- Kempkes, S.N., Slot, M.R., Freeney, S.E. et al. Design and characterization of electrons in a fractal geometry. Nat. Phys. 15, 127–131 (2019).